Key Points
A process has been developed to engineer nanoscale arrays of conducting channels for advanced scalable electronic circuitry
Using ion implantation and lithography, investigators created patterns of topological surface edge states on a topological material that made the surface edges conductive while the bulk layer beneath remained an insulator
Low energy ion implantation, neutron and X-ray reflectometry techniques at ANSTO supported the investigation, which was led by UOW researchers
A large collaboration of researchers led by the has used nuclear techniques at ANSTO and other methods to develop a process to engineer nanoscale arrays of conducting channels for advanced scalable electronic circuitry.
As reported in a in Nature Communications, investigators created patterns of topological surface edge states on antimony telluride (Sb2Te3) that made the surface edges conductive while the bulk layer beneath remained an insulator.
The prepared material is a 3D topological insulator, a new state of matter with insulating energy gaps in the bulk and gapless conducting edge or surface states.
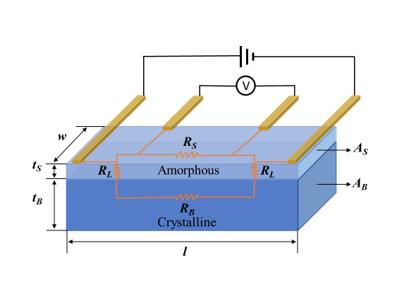
The irradiation displaced atoms causing the transformation of the surface from a crystalline to a disordered glassy state.
“The research answers a fundamental question if crystalline forms of topological materials can be transformed to glassy topological insulators when subjected to strong lattice disorder,” explained ANSTO Instrument Scientist Dr David Cortie, a Research Associate Investigator at the Future Low Energy Technologies (FLEET) Centre, who supervised lead author Abdulhakim Bake.
“Our research shows that, even if transformation may be possible in special cases according to past theory, there is no universal pathway and materials, such as antimony telluride, are instead converted to so-called trivial insulators”.
Previous research by scientists at the established that a topological insulator could function as the ‘on’ state of a transistor with current carried by the conducting edges. This enables them to overcome traditional barriers for electron flow in a transistor.
“The question we addressed may sound a bit academic and uninteresting to people outside the field”, said Dr Cortie.
“But it is rather important, in that, it potentially holds the key to designing and building the trillions of transistors needed for energy-efficient quantum electronics using a nuclear technique.
Ion beam implantation has been commonly used for a very long time to modify the properties of materials by customised doping, for example, the doping of silicon, and is actually the second largest industrial use of accelerators (after medical).
Until recently, however, this approach has not been demonstrated in 2D materials and topological insulators, which are widely considered a promising platform for types of quantum electronics.
In the work by Cortie’s team, lateral patterns were etched on thin flakes of the materials using a focused ion beam (FIB) instrument at the University of Wollongong using gallium ions at low energy (5-30 keV) at varying fluences. The gallium ions penetrate only several nanometres into the material.
The researchers were able to combine ion beams and various forms of lithography to achieve dimensions smaller than 20 nanometres.
The ion irradiation, which was carried out with three types of interfaces under varying temperatures changed the atomic structure and the electronic properties.
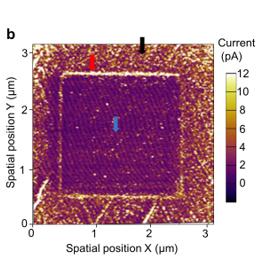
Importantly, the change in surface conductivity occurred at room temperature, suggesting a radical change in the electronic structure. To show this, key conducting atomic force microscopy measurements were performed by Dr Peggy Zhang, who is a Women-in-FLEET Fellow at the UNSW.
X-ray diffraction and cross-sectional transmission electron microscopy confirmed the phase transition and further experimentation with X-ray reflectometry and neutron reflectometry was used to gain insights into optimum ion beam dose to achieve the conversion.
Neutron reflectometry on the newverified the thickness and morphology of the thin films and confirmed that the material remained the same chemical compound after the irradiation.
“The trick here is the judicious choice of the irradiation dose or what we normally call ion fluence. If you under-irradiate, very little happens to the structure, meanwhile, if you over- irradiate, you destroy the material via sputtering. We confirmed that we found the sweet spot for amorphisation,” said Dr Cortie.
The new X-ray reflectometer at the confirmed the orientation and crystallisation of the Sb2Te3 below the nanometre-scale implanted region.
While early work made used of a focused ion beam instrument which is limited to very small sub-millimeter patterned regions, the researchers were later able to demonstrate the approach also worked on large wafer sized area cm2 sized films using a versatile low-energy (1-50 keV) ion implanter (LEII) on the r at ANSTO’s .
“Our implanter is optimised to implant ions in a relatively larger area than the focused ion beam instrument. We implant through a mask to a cover area from millimetres to centimetres,” explained Accelerator scientist Dr Zeljko Pastuovic.
Several different types of material were irradiated, including the antimony telluride, bismuth telluride and bismuth selenide.
“Our system is very flexible, and can run many different types of ions, from light gases to metallic targets.”
The Centre for Accelerator Science supports research with ion implantation on electronic circuits for industrial and space applications.
Collaborating organisations included the University of Wollongong, FLEET, the University of NSW, RMIT, Technical University of Munich, University of Shanghai for Science and Technology, and Monash University.
The Australian Centre for Neutron Scattering has numerous collaborations in the semiconductor/quantum technology sectors, including leading international labs. ANSTO collaborates with Australian companies, such as Quantum Brilliance.
“The question of amorphous and quasicrystalline topological insulators is attracting a great deal of attention within the sector. Our work provides some of the first experiments and should be useful to many people working in this field,” said Dr Cortie.
“On the technological front, I also believe we have unveiled a very useful pathway towards scalable topological electronics utilising ion beams to define surface electronics”.